INTRODUCTION
During the period December 2019–December 2020, the SARS-CoV-2 has infected more than 70 million people and caused far more than 1.6 million deaths worldwide1. SARS-CoV-2 contains monoclonal RNA, has a spherical structure with characteristic corona-like glycoprotein projections, hence the name Corona Virus (CoV), and causes the disease COVID-19 (Corona Virus Disease-19)2-4. SARS-CoV-1, another coronavirus, was responsible for the outbreak of Severe Acute Respiratory Syndrome (SARS) in 2003 and the Middle East Respiratory Syndrome (MERS) in 20075. Although mostly asymptomatic, COVID-19 sometimes presents with mild symptoms, myalgias, taste and smell changes in some cases, or moderate pneumonia. Less commonly, COVID-19 manifests as severe pneumonia and Acute Respiratory Distress Syndrome (ARDS), mainly affecting older patients and people with previous comorbidities and inducing high mortality6. Specific treatment for COVID-19 is currently not available, and its management mainly consists of preventing transmission by avoiding exposure to the virus. The WHO (World Health Organization) recommends adopting hygiene measures, such as frequent and thorough handwashing with soap and water, antiseptics use, smoking cessation, social distancing, and application of face-nose masks7. The recommended treatment for non-critically ill patients includes oxygen, dexamethasone, and remdesivir administration. Dexamethasone appears to increase patients’ survival requiring oxygen therapy or invasive mechanical ventilation, while remdesivir may reduce their hospital stay8,9. Newer antiviral drugs and monoclonal antibodies are under evaluation. In ICU patients with COVID-19 additional hemodynamic and organ support is often necessary5. In intubated and non-intubated patients, the prone position appears useful, while extracorporeal oxygenation (ECMO) benefits selected patients. During their hospitalization, patients with COVID-19 develop secondary bacterial infections. The present article attempts to provide a comprehensive review of the currently available literature on secondary bacterial infections in COVID-19 patients.
REVIEW
Secondary infections in patients with COVID-19
Data on the frequency and characteristics of secondary infections in patients with COVID-19 are limited and conflicting. Initially, studies in China, the United States, and the United Kingdom identified bacterial infections in this group of patients as minor10-13. A retrospective study from the UK included 836 patients with confirmed SARS-CoV-2 infection and a control group of flu patients of the same period (2019–2020). In the first five days of hospitalization of patients with COVID-19, the rate of bacterial infections was 3.2%. This percentage amounted to 6.1% throughout their hospitalization, without reported fungal infections. The results were similar to those of the control group14.
Another retrospective study of 918 patients with COVID-19 from Wuhan, China, found that only 7.1% developed a fungal or bacterial infection, such as pneumonia (32.3%), bacteremia (24.6%), and urinary tract infection (21.5%)15. Also, in 150 hospitalized patients with COVID-19 in Wuhan, China, the secondary bacterial infection incidence was 15%; however, among non-survivors, this rate rose to 50%16. In another study of 41 patients with COVID-19 from Wuhan, only four patients (10%) developed a secondary infection. However, only thirteen patients (32%) entered the ICU, of which six (15%) died17. In another study, the most common complications in 113 non-survivors with COVID-19 were sepsis due to Acinetobacter baumannii or Klebsiella pneumoniae and ARDS10. Respiratory tract infections were reported in 24 of 30 patients with COVID-19 (80%) in Qingdao, China, who developed IgM antibodies to influenza A virus (60%) and influenza B virus (53%), Mycoplasma pneumoniae (23.3%), and Legionella pneumophila (20%). Strikingly, the incidence of identical infections in patients with community-acquired pneumonia from the same geographical area was only 20.90%18. Also, elderly patients (>60 years) with COVID-19 often develop bacterial infections (42.8%)19. The rate of bloodstream infections in ICU reaches approximately 25% after the 15th day of hospitalization and 50% after the 30th day20. The incidence of COVID-19-associated pulmonary aspergillosis (COVID-Associated Pulmonary Aspergillosis – CAPA) varies from 4% to 35%, depending on the type of study (retrospective or prospective)21. Eventually, according to a recent meta-analysis, the prevalence of confirmed bacterial infections in ICU admitted patients with COVID-19 is approximately 14%, without being classified as early or late22.
Pathophysiology of secondary infections in patients with COVID-19
Damage to the respiratory epithelium
The first line of defence of the respiratory system is the mucosal ‘device’ (cilia). The mucus covering the airway epithelium contains mainly glycoproteins (mucins) that support innate immunity through their antiviral and anti-inflammatory properties and allow viruses to be trapped and cleared23-25. After the virus invades the airways, epithelial cells produce the classical interferons (INFs), especially INF-a and INF-b, limiting virus spread26-28. In the airways’ epithelium, microorganisms’ recognition by special receptors occurs, triggering immune reactions by detecting bacterial and viral components. Infection of the airway epithelium by viruses leads to increased expression of platelet-activating factor receptors (PDF-r) and intracellular adhesion molecule-1 (ICΑΜ-1), causing the chemotaxis of macrophages, neutrophils, natural killer cells, and eosinophils from the bloodstream to the site of infection29.
Dysregulation of the immune response
Viral infections interfere with mucosal clearance, resulting in increased adhesion of bacteria to the mucosa and bacterial colonies’ formation. Excessive mucus concentration (as a reaction to viral infection) also inhibits the accumulation of immune cells and antibacterial molecules30. Besides, cell death impairs pathogenic microbes’ mechanical clearance and promotes the emergence of new receptors for bacterial adhesion or leads to biofilm formation, mainly by Str. pneumoniae31,32. Increased bacterial adhesion, caused by increased surface receptor expression (e.g. by PAF-r involved in the penetration of Str. pneumoniae into the respiratory epithelium) and by desensitized Toll-Like Receptors (TLRs) of lung cells (due to the action of viruses), contributes to the development of secondary bacterial infection33. The TLR4 and TLR5 channels change in shape, resulting in decreased neutrophil chemotaxis and increased bacterial adhesion, such as Str. pneumoniae and Pseudomonas aeruginosa, in the airway epithelial cells34,35. Other bacteria such as S. aureus are incorporated into the respiratory epithelial cells and cause a further increased ICAM-1 expression36.
On the other hand, activation of TLRs induces the synthesis of INFs, which enhance the inflammatory response. The interaction of viruses and host cells leads to the secretion of inflammatory cytokines (e.g. TNF-a), which despite their bactericidal activity, can be harmful, causing the appearance of a hyperinflammatory reaction (cytokine storm), characterized by increased mortality26,35,37. The risk of secondary infections is higher in patients with hypoxemic respiratory failure (with or without severe lymphopenia) who need ICU admission in the early stages of the disease (<48 hours) (Table 1). Advancing age, comorbidities, concomitant administration of immunosuppressive agents, or increased inflammatory markers (C reactive protein and ferritin) are not substantial risk factors for secondary infections38.
Treatment of secondary infections in patients with COVID-19
Patients with COVID-19 develop co-infections (bacterial infections that develop simultaneously with the viral infection) and superinfections (bacterial infections that develop during hospitalization). These infections overlap with COVID-19 disease in clinical and laboratory findings and imaging, requiring increased surveillance for their early diagnosis, frequent cultures, and measurement of biomarker levels (e.g. procalcitonin)19,39. Table 2 shows the algorithm proposed for the diagnostic approach of these infections.
About 15% of patients with influenza manifest bacterial co-infections, with the most identified bacteria being Str. pneumoniae, Staph. aureus and Haemophilus influenzae. Patients with COVID-19 are less likely to develop community-associated infections (approximately 8.4%), and the responsible bacteria’s incidence is unknown. In patients with COVID-19 and co-infection, the treatment protocols of community-associated infections are applied, emphasizing Str. pneumoniae infections with the administration of a β-lactam in combination with a macrolide. In Greece, the resistance rate of Str. pneumoniae to penicillin is low (about 5.6%); however, macrolide is highly resistant (about 40– 45%).
Inpatients with COVID-19 experience infections caused by nosocomial pathogens (Klebsiella pneumoniae, Pseudomonas aeruginosa, bacteria that produce broad-spectrum β-lactamases, Acinetobacter spp, E. coli, Enterococcus spp). Simultaneously, small studies from China reported polymicrobial infections caused by Candida albicans, Acinetobacter baumannii, Klebsiella pneumonia, and Aspergillus fumigatus17,15,40,41. The onset of secondary infections depends on patients’ comorbidities, use of invasive techniques (e.g. central venous lines placement, hemodialysis techniques) leading to injury of the anatomical defensive barriers (e.g. skin), colonization of the patients, compliance with hand hygiene measures and nurse/patient ratio.
In patients with COVID-19, antimicrobial therapy can be empirical or targeted. The choice of empirical treatment should consider the local epidemiology and antimicrobial resistance profile. The early choice of an appropriate (right drug) and adequate (right dose) antibiotic treatment assumes the knowledge of the local epidemiology (data from the records of the Hospital Infection Committees) and PK/PDs of the selected antibiotics10,15,42,43. Subsequently, the physician can de-escalate or escalate the treatment according to the cultures’ results and the antibiotics’ susceptibility tests42,43. Targeted treatment choice should consider the cultures’ results (bacterial species), the susceptibility tests, and Minimum Inhibitory Concentration (MIC) of each antibiotic. Procalcitonin levels distinguish between bacterial and viral infection and serve to discontinue or continue antibiotic treatment41,43,44.
Often, the local epidemiology differs not only from country to country but also among the same geographical area’s hospitals. For this reason, the treatment of secondary infections in patients with COVID-19 cannot follow specific treatment protocols. In China, cephalosporins, carbapenems, and quinolones have been the first-line antibiotics for the treatment of infections, along with the administration of antiviral agents41. However, in Greece, the treatment must be different because of the increased incidence of Multi-Drug Resistant (MDR) strains, emphasizing the National Treatment Guidelines for these infections. Besides, the adequate dose of an antibiotic (adequate treatment) depends on specific characteristics of the treated patient (e.g. increased body mass index, increased volume of distribution in septic shock due to capillary leakage, hypoalbuminemia, and renal dysfunction, leading to low concentrations of antibiotics at the site of infection), and the profile of antimicrobial resistance. The optimal dose of an antibiotic also depends on its pharmacokinetic/ pharmacodynamic properties (PK/PDs) (antibiotic dose- or time-dependence, the volume of distribution, augmented creatinine clearance) (Figure 1), targeting in therapeutic concentration at the infection’s site without concomitant toxicity. Therapeutic Drugs Monitoring (TDM) is the method to achieve therapeutic concentrations of an antibiotic by measuring its trough levels (minimum concentration before the next dose). The continuous infusion (within 24 hours) of the total daily dose of an antibiotic (mainly β-lactams) helps to steadily obtain adequate levels of antibiotic concentration (above MIC) at the site of infection (Figure 2)45.
Figure 1
Main pharmacodynamic parameters of antibiotics. AUC (Area Under the Curve) = area enclosed between Cmax and MIC, indicating the concentration of antibiotic at the site of infection. Cmax (Concentration maximum) = maximum concentration achieved after a dose of antibiotic, MIC (Minimum Inhibitory Concentration) = minimum inhibitory concentration required to fight a bacterium, t (time) = the time at which an antibiotic displays concentrations above MIC
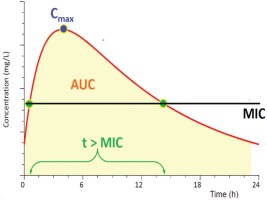
Figure 2
Intermittent, prolonged and continuous administration of antibiotics. (A) Intermittent administration of antibiotics. In susceptible pathogens (f.i. MIC 0.25 mg/L), the concentration of antibiotic at the site of infection (AUC1), and the time of concentration above MIC [fT > MIC(0.25mg/L)] are adequate. When the same pathogen is resistant (f.i. MIC 8 mg/L), the same parameters [AUC2, fT > MIC(8mg/L)] are reduced. (B) Prolonged (blue line) and continuous (red line) administration of antibiotics. AUC3 and fT > MIC(8mg/L) are increased in relation to intermittent administration. Continuous administration shows continuously increased values of the same parameters45
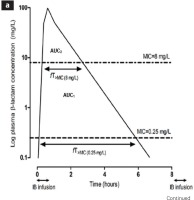
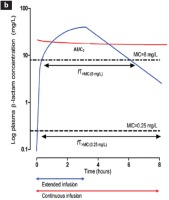
CONCLUSION
Data on the incidence and characteristics of secondary infections in patients with COVID-19 are currently limited. The risk of bacterial infections increases in patients with COVID-19 admitted to the ICU. The innate immunity of patients with SARS-CoV-2 is impaired, leading to the development of secondary bacterial infections. According to local epidemiology and the antimicrobial resistance pattern, a key issue is choosing an appropriate empirical treatment with broad-spectrum antibiotics. The early, appropriate, and adequate treatment reduces mortality, while of great importance is the de-escalation according to cultures’ results and isolated pathogens’ susceptibility.